In this article, we’ll give you some tips on how to use a molecular model for learning chemistry.
Nothing can beat a nice molecular model kit for leaning 3D visualization of molecules. In this guide you will learn how to use it, and for what it can be helpful.
If you don’t have one yet, check out our molecular model kit buying guide and find the best one for you!
Molecular Geometry and Covalent Bonding
Molecular models are usually used in organic chemistry classes, but their utility is not limited to o-chem. Some important general chemistry concepts that can be better understood with a model are molecular geometry and covalent bonding.
A cool example is using it to identify stereoisomers of inorganic or organometallic metal complexes:
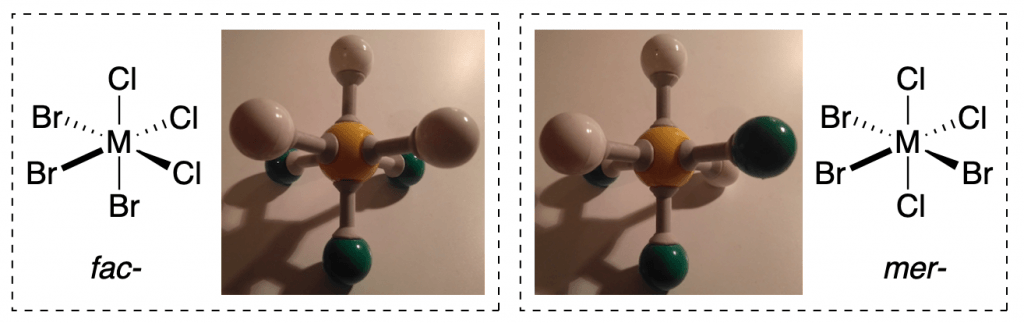
Most standard kits come with a variety of atoms with different numbers of shareable valence electrons, which are represented as holes. VSEPR theory assumes that the geometry around each atom depends on those valence electrons repelling each other. Because of this, you can get hands-on experience with many different geometric configurations using a molecular model kit.
Unlike ionic bonds, which are formed by the donation and acceptance of electrons, covalent bonds are the result of electrons being shared between two atoms in a molecule. The sharing is not necessarily 50/50, but even so, the bond pieces used in model kits are a good visual and tactile reminder of that shared electron pair.
In any case, these are great for grasping some very important basic concepts in chemistry.
Organic Nomenclature: Build it with Your Molecular Model!
While not normally necessary, model kits can help you with some intentionally tricky organic nomenclature questions that sometimes show up on exams.
You might know that your first step in naming an organic molecule is to find the longest uninterrupted carbon chain. Where they try to trick you on exams is drawing the structure out in a way that makes the longest chain counterintuitive.
Practicing with models can help you learn to look for the longest chain where you might not necessarily expect to find it in the drawing. Physically holding the molecule in your hand allows you to look at it from every angle so that you don’t get tricked.
Alkanes, Alkenes and Alkynes
In organic chemistry, molecular model kits are very useful for understanding some of the properties of double and triple bonds. However, they do have important limitations here, and you need to be aware of them as you learn chemistry.
If you take a look at a carbon atom in your kit (usually black), you’ll see that it has four holes where you can attach bonds, representing carbon’s four valence electrons. When carbon is bonded to four things via single bonds, each of those bonds is a hybrid of s– and p-orbitals; in this case, since all four bonds are equivalent, they must each have sp3 hybridization (one part s and three parts p, divided into four equal bonds), and we call these sigma bonds. (Pop quiz: what geometry is this? What are the bond angles?)
As soon as you make double bonds, things begin to change. The model kit comes with longer flexible bonds that are meant to be used to form double and triple bonds. Looking at a model of, say, 2-butene, it is immediately clear that there are no longer four equivalent bonds on carbons 2 and 3; you had to use different pieces to even represent those bonds on your model.
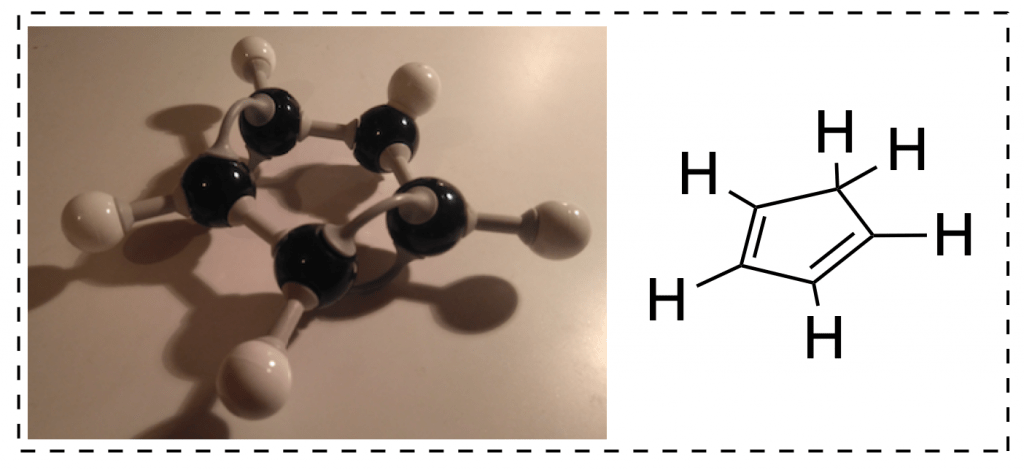
What might not be clear from the double bonds is that the flexible bond is actually a representation of the pi bond that is left.
Hybridized Orbitals, Sigma and Pi Bonds
Carbon is now bonded to three things, one of which is attached via a double bond. Just like we used one part s and three parts p to create four sp3 orbitals before, we now will take one part s and two parts p to make three sp2 hybridized orbitals (again, take note of the geometry and bond angles). These three all form sigma bonds. What about that leftover p? That is the second long, flexible piece in your double bond—it is a purely p orbital, and the bond it forms is called a pi bond.
The same goes for a triple bond: carbon is bonded to two things, so you take one part s and one part p to make two sp hybridized orbitals (sigma bonds), and your two leftover p orbitals each form a pi bond. Thus, a carbon-carbon triple bond is made up of one sigma bond and two pi bonds.
Now, pick up your model of any alkane you like, and notice how all of its bonds have free rotation. Next, make a model of the corresponding alkene or alkyne, and try to rotate about the double or triple bond. You can’t, right? There is no rotating about that bond now without first breaking a bond. This is a very important concept to grasp in organic chemistry, and it is one that molecular models illustrate beautifully.
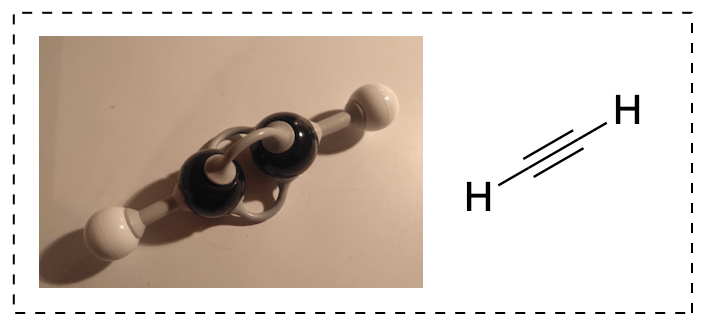
The drawback to visualizing single, double and triple bonds with some model kit is that the lengths of the bonds may not be to scale. The Molymod one of the pictures comes fairly close to reality, in the sense that triple bonds look shorter than double bonds, but it is not always the case.
You’ll just have to remember that, even though it might look like there is more space between atoms in your double bond, this is just the way the pieces are made. In reality, single bonds are the longest (154 pm), followed by double bonds (134 pm) and then triple bonds (120 pm).
Visualizing Chirality and Stereochemistry with a Molecular Model
Chirality is one of the trickiest concepts for first-semester organic chemistry students, and a molecular model kit can really make it “click”.
On paper, it can be hard to see the difference between, say, (R)-1-chloroethanol and (S)-1-chloroethanol. But as soon as you build the two models—nonsuperimposable mirror images of each other—you will see that, just like your right hand and your left hand, they are not the same.
Determining the R/S configuration around chiral carbons is almost always easier when you can hold a physical model and turn it over in your hands. No matter how the molecule is presented to you on paper (dashes and wedges, sawhorse, Fisher projection, Newman projection, etc.), a molecular model lets you see it in 3D and orient it correctly.
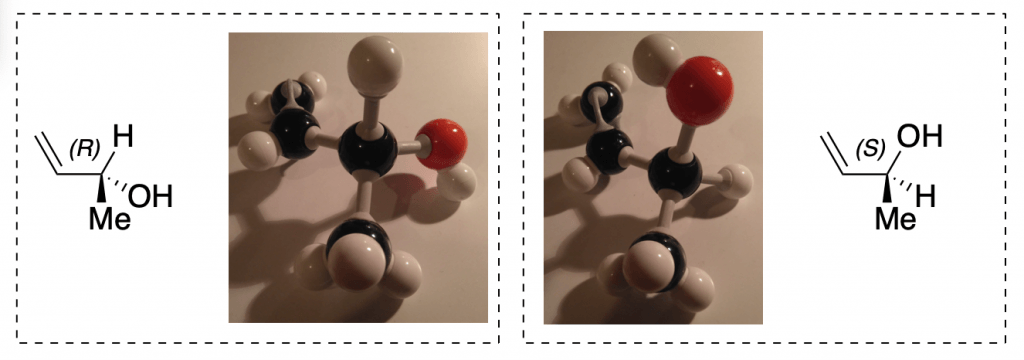
However, being able to rotate different projections and determine R/S configuration without a tactile aid may be necessary if a molecular model kit is not allowed on any exam you might take. In this case, definitely practice doing it completely on paper, but take advantage of the model as a tool to help bridge the gap in spatial reasoning if you find this challenging.
Another fabulous use for molecular models on this topic is demonstrating how a molecular can have chiral carbons and still not be a chiral molecule.
For example, use your kit right now to build cis-1,2-dichlorocyclohexane, confirm that it does indeed have two chiral centers, and then build its mirror image. You will see that the mirror image is superimposable, and therefore, the molecule is not chiral.
Go ahead and try the same but with trans-1,2-dichlorocyclohexane. What happens on this case? Is that molecule chiral or not? Hint: look for mirror planes.
Steric Hindrance and Reactivity
The last topic we’ll cover in this post on how to learn chemistry using a molecular model kit is steric hindrance. This is a key concept in understanding organic reactions because it will help you see whether a given nucleophile or electrophile is accessible enough to participate in the reaction.
To see this, all you need to do is build a primary, a secondary and a tertiary substrate. In our case, we will show you the difference in reactivity through nucleophilic substitution reaction of two chlorophosphines.
You know that an atom bond to a good leaving group such as a halide (chloride) is an electrophile and, in theory, vulnerable to nucleophilic attack. But do you see how much “stuff” would get in the way of your nucleophile if it tried to attack an electrophilic center with a tert-butyl group? That is steric hindrance, and it can make the difference between a straightforward reaction and one that is a total nonstarter.
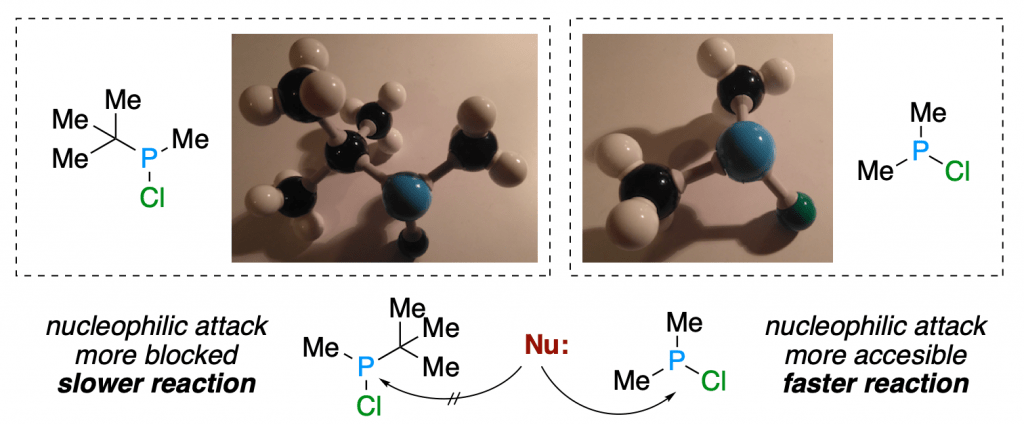
You can clearly see how the presence of a bulky group really hinders the approach of an external nucleophile (too much repulsion!) and blocks the reactivity, sometimes slowing down the reaction, and sometimes shooting it down completely.
If you are interested, I recommend you to take a look to our previous post on steric effects in organic reactions to learn more.
Apart from reaction rates. using your molecular model kit to illustrate the steps in substitution and elimination reactions is also helpful in predicting the stereochemistry of the products.
Choosing the Best Molecular Model Kit
Those are just some of the many ways a molecular model kit can help you learn chemistry, especially organic chemistry. Real molecules exist in three dimensions, and you will get a better understanding of what’s really going on with them when you can hold them in your hand, manipulate them, and look at them from every angle. The pictures from this guide were made using a Molymod molecular kit, since it was the only one I had around when I prepared the post.
However, if you want to make sure you buy the best molecular model kit, we highly recommend Dalton Lab Kit.
It’s middle of the road in terms of price, but with 306 pieces it is an excellent value. Plus, it comes with access to the manufacturer’s online 3D molecular modelling software. You also get the peace of mind of a money back guarantee.
Dalton Labs Molecular Model Kit
But if you decide to buy a different kit, no worries!
We have an entire review about choosing the best molecular modeling kit to fit all your needs: Check our complete review here!
They are very affordable and worth every penny—a molecular modelling kit is one of the best investments you can make in your chemistry education.
this is very informative article for me. thank you